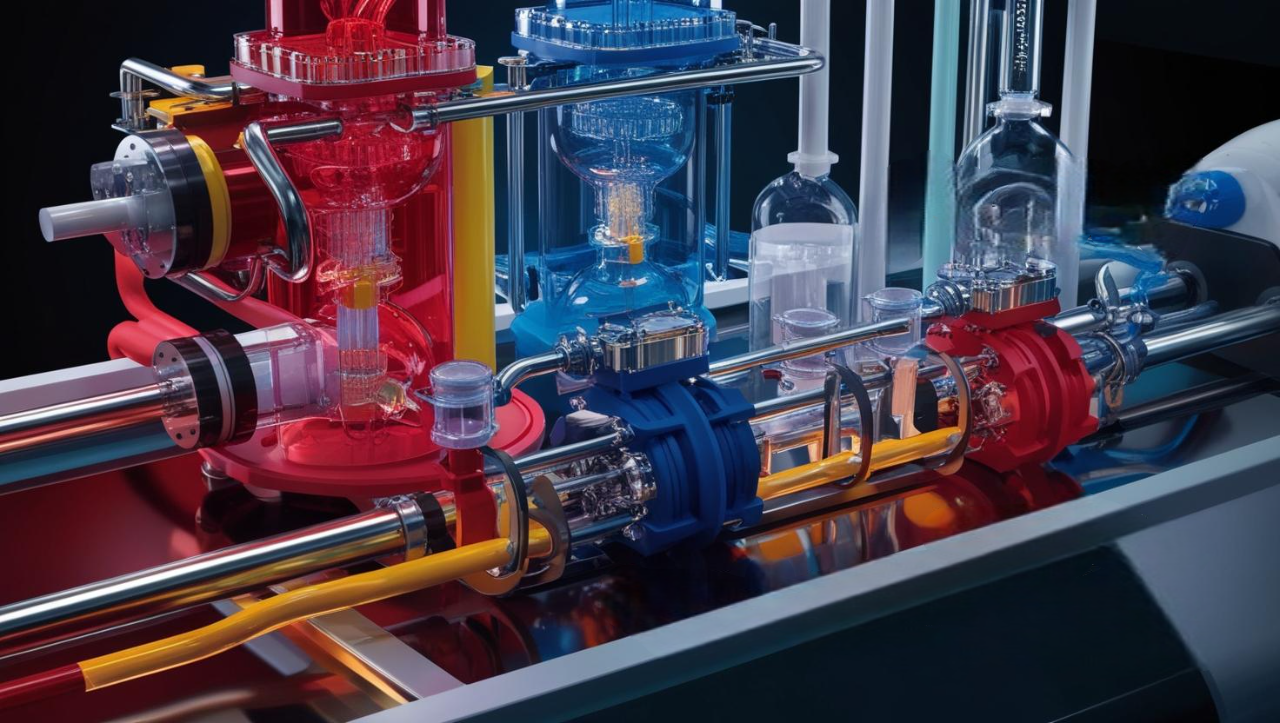
Introduction to Biocatalysis
Biocatalysis is all about using nature’s tiny powerhouses—enzymes and whole cells—to drive chemical reactions. Unlike traditional chemical catalysts that rely on harsh conditions and heavy metals, biocatalysis is a cleaner, greener, and highly specific alternative. Thanks to enzymes’ ability to selectively convert substrates with minimal waste, industries are increasingly turning to biocatalysis for a more sustainable future.
Why move from batch to flow biocatalysis?
While biocatalysis has a lot going for it, scaling it up for industrial applications isn’t always smooth. Batch biocatalysis struggles with enzyme instability, inconsistent yields, and reaction inefficiencies. Problems like product inhibition and enzyme deactivation make it hard to maintain long-term, high-yield production. That’s where flow biocatalysis comes in. This continuous processing method allows for better enzyme reusability, real-time monitoring, and improved scalability—making it the clear winner over batch systems for large-scale operations.
Understanding Flow Biocatalysis
Figure 1: Flow Biocatalysis overview
Flow biocatalysis keeps things moving—literally. Instead of performing reactions in a static batch, reactants continuously flow through reactors packed with immobilized enzymes. This setup allows for more precise reaction control and higher efficiency. The flexibility of flow systems means that reactors can be arranged in series or parallel, making multi-step reactions much more manageable. Need to swap out an enzyme module? No problem! Lower-stability enzymes can be replaced on the go, keeping the process running smoothly.
Automation plays a massive role here too. AI-driven predictive modeling, in-line monitoring, and real-time adjustments help optimize reaction conditions without human intervention. This makes processes more consistent, reduces waste, and improves overall efficiency.
Advances Driving Flow Biocatalysis
1. Smarter Enzymes with AI and Computational Tools
With machine learning and molecular simulations, scientists can fine-tune enzymes for better stability and efficiency. Advanced computational models help identify beneficial mutations without endless trial-and-error experiments, leading to more robust biocatalysts that can handle industrial demands.
2. Enzyme Immobilization for Better Performance
Attaching enzymes to solid supports (via covalent binding, adsorption, or encapsulation) makes them last longer, reduces waste, and improves recyclability. Advanced nanomaterials and hybrid carriers further boost mass transfer and catalytic activity, cutting down costs and increasing efficiency.
3. Modular Flow Reactor Design: Types of Flow biocatalytic setups
The design of flow reactors has become increasingly modular, enabling scalability and adaptability. Packed-bed reactors, microreactors, and 3D-printed reactors are being developed to accommodate immobilized enzymes for various applications. These designs allow for seamless scaling by connecting multiple modules in series or parallel, meeting industrial scale demands.
Semi-Continuous Flow Pumps
Semi-continuous flow pumps allow periodic reactant addition while maintaining a steady state, making them ideal for oxidation-reduction and hydrolysis reactions that require enzyme regeneration or staged substrate feeding. This controlled dosing prevents enzyme deactivation, improves reaction efficiency, and extends operational lifetime.
True Continuous Flow Pumps
True continuous flow pumps provide an uninterrupted flow of reactants and products, ensuring consistent reaction conditions. They are essential for large-scale industrial processes such as transaminations, esterifications, aminations, and acylations, where stable reaction environments maximize yield, scalability, and efficiency.
Figure 2: Continuous Flow Biocatalysis Systems. Illustration of various continuous flow setups used in biocatalysis.
There are two types of pumps integrated into the basic flow system (a) Continuous Flow System, and (b) Advanced Flow System. The basic flow system typically relies on semi-continuous pumps for controlled reactant addition and product removal. The continuous flow system employs true continuous pumps for uninterrupted processing, enhancing efficiency in large-scale operations. The advanced flow system integrates both pump types, adapting to reaction complexity and automation requirements to optimize process control and scalability.
Figure 3: Types of flow systems
Inching towards industrial applications
Flow biocatalysis isn’t just making headlines in research circles—it’s getting real in industry. From cosmetics and pharmaceuticals to biofuels, wastewater treatment, and specialty chemicals, many of the technologies and examples now gaining attention are already being scaled up or piloted in industrial environments.
One of the most exciting spaces?
Pharmaceuticals—where flow biocatalysis is streamlining the synthesis of chiral intermediates and APIs.
Turning Enzymes into Flow: A Smarter Way to Make Chiral Amines
Immobilized enzymes are shaking up how we approach chiral amine synthesis—and the latest research proves just how powerful they can be when teamed with continuous-flow systems.
In a recent study, Molnár et al. explored the potential of (S)- and (R)-selective transaminases (TAs) to resolve a range of racemic amines. While many of these substrates have been tested before, the resolution of rac-1d (3,4-dimethoxy-α-methylbenzylamine) marked a new milestone—it was tackled with TAs for the first time ever.
Figure 4: Whole cells were immobilised on silica sol-gel matrix which was further set up in batch and flow mode for the production of chiral amines.
In the traditional batch setup, AtR-TA (Aspergillus terreus) emerged as a top performer for (R)-selective resolution. It delivered solid enantioselectivity—above 99% ee in some cases—and moderate to good conversions. But the performance wasn’t always predictable. For example, the resolution of rac-1c (1-phenylethylamine) in batch only reached 72.9% ee, hinting at challenges like enzyme instability and inconsistent reaction dynamics. Simply put, batch mode had its limits.
That’s where flow chemistry came in—and changed everything.
By moving to a continuous-flow setup, the researchers unlocked better control, better efficiency, and most importantly, more consistent enantiomeric excess across the board. For the same rac-1c, ee jumped to 98.8%, and conversion also improved slightly. The newly explored rac-1d reached 53.5% conversion and 99.2% ee—a remarkable outcome for a first-time substrate.
Beyond the numbers, flow chemistry brought speed to the table. Reactions that once took hours—or even days—were now wrapping up in minutes. The space-time yield (STY) for AtR-TA hit 42.2 mg/cm³/day, and the enzyme stayed active for over 21 hours, a big leap from the batch setup.
Table 1: Comparative performance of AtR-TA in batch and flow modes across different racemic amine substrates, highlighting conversion efficiencies and enantiomeric excess (ee%). (rac-1a: 2-aminoheptane, rac 1b: 1-methyl 3-phenylpropylamine, rac 1c: 1-phenylethylamine, rac 1d: 3,4 dimethoxy-alpha-methylbenzylamine)
This study is a great example of how continuous-flow biocatalysis isn’t just a lab trend—it’s a scalable, reliable, and time-saving strategy for producing enantiopure compounds. With enzymes like AtR-TA delivering consistent results and staying stable for extended periods, the future of sustainable chiral synthesis is already in motion.
Challenges and Opportunities
Despite its advantages, flow biocatalysis faces challenges, including:
- Enzyme Stability: Improving enzyme robustness under continuous operation is crucial. There is enzyme leaching after washing steps of immobilized enzyme, enzyme denaturation due to high concentration of solvents used in chemo-enzymatic processes.
- Cost of Setup: The same rules do not apply which were used in the batch biocatalysis, hence high initial costs for specialized flow reactors and immobilization techniques are seen.
- Integration: Seamlessly integrating flow biocatalysis with existing industrial processes requires further advancements in automation and compatibility. In-line analytical techniques, innovative apparatus better suited for hybrid processes which also makes downstream processing easier.
However, the opportunities far outweigh these challenges. Emerging areas like hybrid chemoenzymatic processes, biofuel synthesis, and green polymer production present exciting growth avenues for flow biocatalysis. Additionally, innovative methods such as Pickering emulsions, microwave and ultrasound-assisted systems, and microfluidic flow reactors are advancing the field by enhancing reaction efficiency, improving mass transfer, and facilitating better enzyme-substrate interactions, ultimately making processes more sustainable and scalable.
Conclusion
Flow biocatalysis is revolutionizing industrial bioprocessing by offering continuous, high-efficiency reactions with minimal waste and superior enzyme reusability. From pharmaceuticals to specialty chemicals, industries are rapidly adopting this technology to optimize production and reduce environmental impact.
Looking ahead, AI-driven modeling, smart reactors, and novel biocatalytic approaches will push flow biocatalysis even further. As industries race toward sustainability and cost-effective production, this technology is set to play a leading role in reshaping chemical and pharmaceutical manufacturing. The future of flow biocatalysis isn’t just bright—it’s unstoppable!
References
- Britton J , Majumdar S , Weiss GA . Continuous flow biocatalysis. Chem Soc Rev. 2018 Jul 30;47(15):5891-5918. doi: 10.1039/c7cs00906b. PMID: 29922795; PMCID: PMC6441135.
- Benítez-Mateos, A. I., Contente, M. L., Roura Padrosa, D., & Paradisi, F. (2021). Flow biocatalysis 101: design, development and applications. Reaction Chemistry and Engineering, 6(4), 599 - 611. DOI: 10.1039/D0RE00483A
- Ruscoe, Rebecca & Cosgrove, Sebastian. (2024). Future directions in flow biocatalysis: The impact of new technology on sustainability. Current Opinion in Green and Sustainable Chemistry. 49. 100954. 10.1016/j.cogsc.2024.100954.
- Crotti, Michele & Robescu, Marina & Bolivar, Juan & Ubiali, Daniela & Wilson, Lorena & Contente, Martina. (2023). What’s new in flow biocatalysis? A snapshot of 2020-2022. 3. 1154452. 10.3389/fctls.2023.1154452.
- Romero-Fernández M, Paradisi F. Protein immobilization technology for flow biocatalysis. Curr Opin Chem Biol. 2020 Apr;55:1-8. doi: 10.1016/j.cbpa.2019.11.008. Epub 2019 Dec 19. PMID: 31865258.
- Woodley JM. Accelerating the implementation of biocatalysis in industry. Appl Microbiol Biotechnol. 2019 Jun;103(12):4733-4739. doi: 10.1007/s00253-019-09796-x. Epub 2019 May 2. PMID: 31049622.
- Molnár, Zsófia & Farkas, Emese & Lakó, Ágnes & Erdélyi, Balázs & Kroutil, Wolfgang & Vértessy, Beáta & Paizs, Csaba & Poppe, Laszlo. (2019). Immobilized Whole-Cell Transaminase Biocatalysts for Continuous-Flow Kinetic Resolution of Amines. Catalysts. 9. 10.3390/catal9050438.